
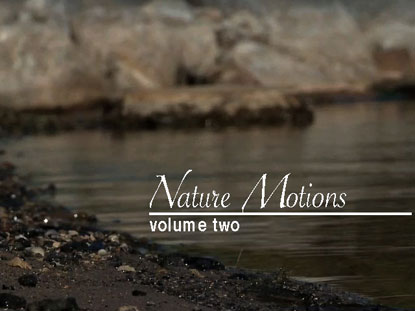
To this end, objective measurements are critical because camouflage evolved to exploit perceptual clustering by observers, so as to fool them 22, 23. Here we improve on these techniques and report a new complementary analysis to describe quantitatively the space, dynamics and reliability of camouflage patterns and, through this, gain insights into its control system. We recently developed methods to track the instantaneous expansion state of tens of thousands of chromatophores in the behaving cuttlefish S.
#Nature motion background skin#
The generation of a skin pattern therefore results from the appropriate coordination and control of tens of thousands of motoneurons by a system that interprets complex visual scenes 2, 3, 18. The expansion state of each chromatophore depends on a radial array of muscles controlling the size of a central pigment sac 21 and, therefore, on the activity of one to a few motoneurons, the dendrites and somata of which lie in the animal’s central brain 6, 7. The generation of 2D skin patterns relies on a motor system that controls the expansion state of up to several million pigment cells (chromatophores) embedded in the animal’s skin 5, among other specialized cell types 17, 20. These sophisticated operations are carried out instinctively 18 by the brain of animals that diverged from us more than 550 million years ago 19, well before large brains existed. Pattern matching does not consist of a faithful reproduction of the substrate’s appearance but, rather, of the visually initiated statistical estimation and generation of that appearance 5. We studied here the 2D features of camouflage and therefore refer to them as skin patterns and to the process as pattern matching.
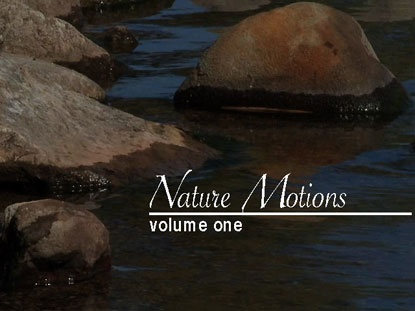
Although both components are technically textural 4, 15, 16, in this field the term ‘texture’ is often applied only to 3D features, caused, for example, by the contraction of skin papillae 5, 17. Pattern motion during blanching was direct and fast, consistent with open-loop motion in low-dimensional pattern space, in contrast to that observed during camouflage.Ĭephalopod camouflage consists of matching the animal’s appearance to that of its substrate and typically contains two-dimensional (2D) and three-dimensional (3D) components. Finally, we compared camouflage to blanching, a skin-lightening reaction to threatening stimuli. Components could also be differentiated by their sensitivity to spatial frequency. However, their identities varied even across transitions between identical skin-pattern pairs, indicating flexibility of implementation and absence of stereotypy. These components varied in shapes and sizes, and overlay one another. Chromatophores could be grouped into pattern components on the basis of their covariation during camouflaging.
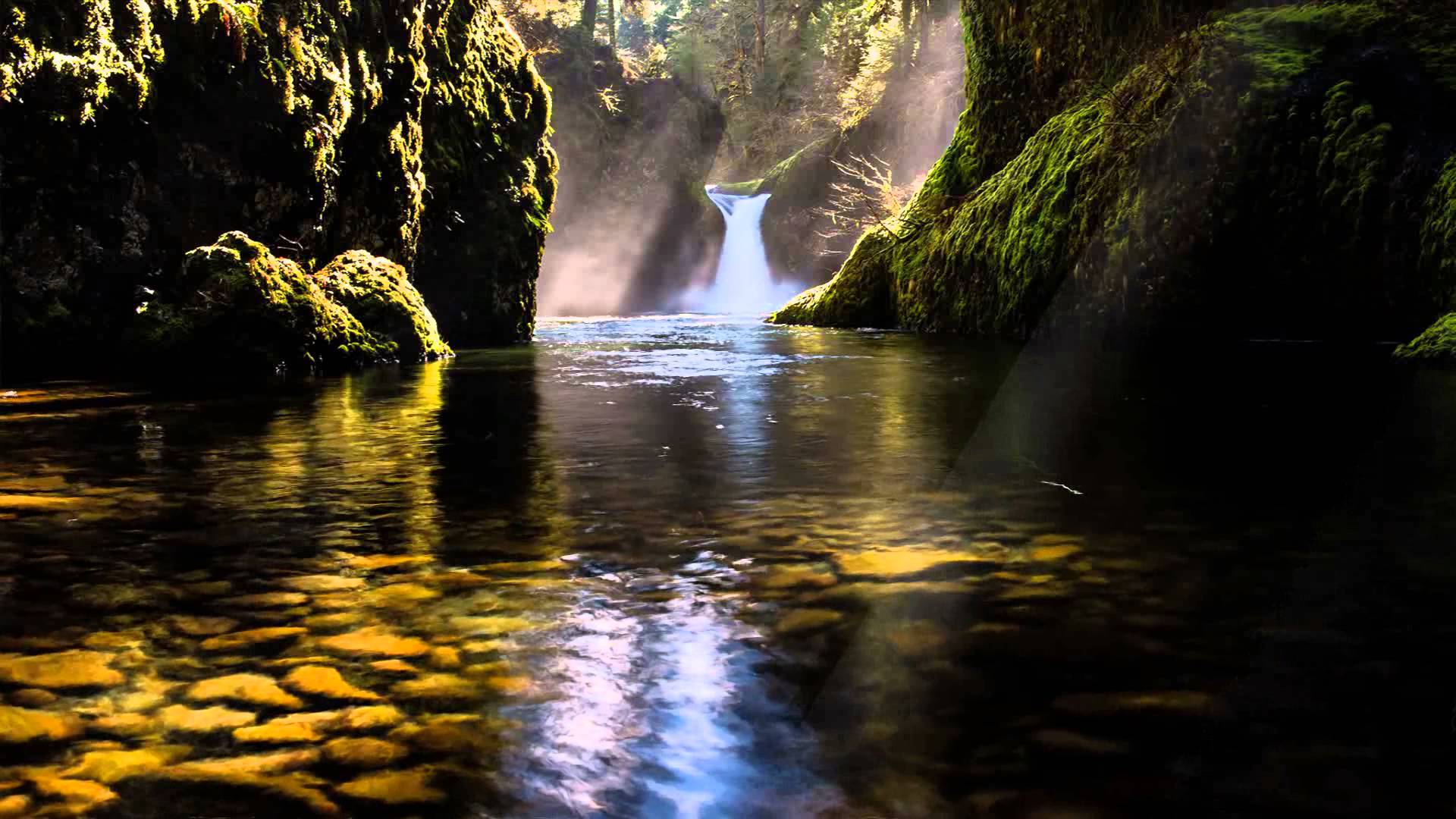
An analysis of hundreds of thousands of images over natural and artificial backgrounds revealed that the space of skin patterns is high-dimensional and that pattern matching is not stereotyped-each search meanders through skin-pattern space, decelerating and accelerating repeatedly before stabilizing.
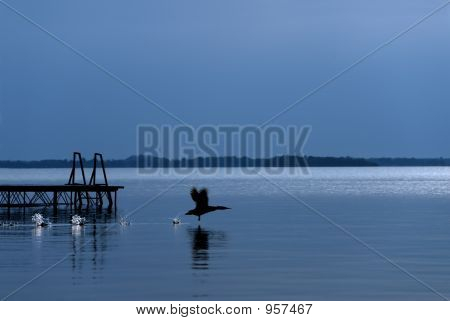
Here, using quantitative methods 14, we studied camouflage in the cuttlefish Sepia officinalis as behavioural motion towards background matching in skin-pattern space. Behavioural experiments also indicated that, although camouflage requires vision, its execution does not require feedback 5, 12, 13, suggesting that motion within skin-pattern space is stereotyped and lacks the possibility of correction. Analysis of cuttlefish images proposed that camouflage patterns are low dimensional and categorizable into three pattern classes, built from a small repertoire of components 8, 9, 10, 11. This behaviour relies on a visual assessment of the surroundings, on an interpretation of visual-texture statistics 2, 3, 4 and on matching these statistics using millions of skin chromatophores that are controlled by motoneurons located in the brain 5, 6, 7. Many cephalopods escape detection using camouflage 1.
